Genomic Stimulus
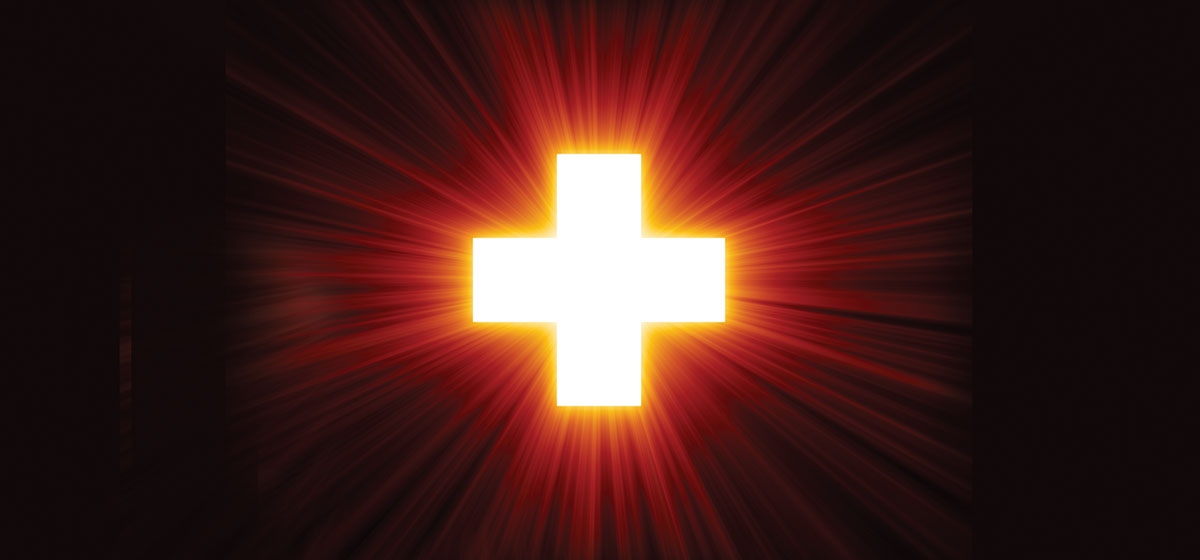
Not long ago, one of the nation’s most dreaded diseases was polio, paralyzing and sometimes killing its victims. Fortunately, polio proved no match for medicine. Just as polio reached its peak in 1952 with 57,000 new cases, a University of Pittsburgh team, led by Dr. Jonas Salk, was testing a vaccine.
Soon, polio all but disappeared in the U.S. and the Salk team accomplished one of the greatest achievements of the 20th century.
While modern medicine has had incredible success—we are living longer than ever—it still falls short. Each year, children are born with genetic conditions that have no cure. And eradicating today’s widespread diseases that typically hit later in life—cancer and Alzheimer’s for example—has so far eluded medicine.
Confronting such complex diseases may require a new, more personalized, approach. Enter the age of genomics, the window into the workings of genes and all cellular pathways underlying disease.
Tinkering with these pathways, unique to every person, is at the heart of what’s being called personalized medicine.
The genomics era got a major boost a decade ago when scientists finally had a copy of the entire DNA blueprint—all 6 billion chemical units of genetic instruction (in other words, the genome) that make us human. This blueprint was the result of a 13-year international effort known as the Human Genome Project. When the first draft was revealed in 2000, then-President Bill Clinton proclaimed this would “revolutionize the diagnosis, prevention and treatment of most, if not all, human diseases.” The British tabloid, The Daily Mirror, proclaimed “It’s one small piece of man… one giant leap for mankind.”
Although the accomplishment has yet to live up to its potential, aspects of personalized medicine are beginning to happen today. “I really, truly, believe that human genomic sequencing [mapping the order of the chemical units in a stretch of DNA] is going to change the medical world, and we’re very well positioned to embrace that future,” said Michael Passineau, Ph.D., director of the Gene Therapy Program at Allegheny General Hospital’s Allegheny-Singer Research Institute.
The Pittsburgh region didn’t play a direct role in the Human Genome Project, but it is a major force in a worldwide effort that stands to advance medicine. Contributing to this effort—not to mention jobs—are several biosciences companies, as well as research powerhouses such as Pitt, UPMC and Carnegie Mellon University. On the North Side, there’s the Allegheny-Singer Research Institute (part of West Penn Allegheny Health System) and on the South Side, the National Resource for Biomedical Supercomputing (See “Beyond Gigabytes” sidebar).
Perhaps no one in Pittsburgh remembers the decoding of the human genome better than M. Michael Barmada, Ph.D. He has devoted his career to gene mapping; studying the genetic variations at the root of human traits, such as eye color, as well as disease. He directs the Center for Computational Genetics, part of the Department of Human Genetics in Pitt’s Graduate School of Public Health. “When the Human Genome Project was finished, we had a lot of hope,” Barmada said. “Some people said, ‘Oh, we’ll have all the answers now’, but we knew that wasn’t going to be the case.”
It turns out that the mapping of the human genome brought forth many surprises and revealed that people, even at the cellular level, are incredibly complex. “The Human Genome Project taught us that we’re only just beginning to understand the genome,” Barmada said. Predicting or curing disease, he adds, requires knowing the variations in our genetic code—those differences that we inherit or that happen over a lifetime. “We were hoping that genetic changes causing inherited diseases would be easy to find. Unfortunately, we discovered there’s a whole lot of differences in the genome that we don’t understand fully.
“The next great frontier is being able to use what we’ve found in genetics to impact medical care. I hope that sometime in the next 10 years we’ll work out a framework to get genetic information to physicians to use in clinical practice. In the not-too-distant future, I think it very likely that people coming to the hospital will have their whole genome sequenced as part of the regular diagnostic process.”
Sequencing a person’s entire genome still belongs mainly to the province of research, but in a few cases, genomes are being sequenced to help diagnose mysterious rare diseases and treat patients. About 30,000 human genomes were expected to have been sequenced by the end of 2011, according to the journal Nature. That number is expected to rise to millions in a few years.
Driving this are plummeting costs. Whole-genome sequencing went from $8.9 million in July 2007 to about $10,500 last July. In January, CMU alumnus Jonathan Rothberg announced that his company, Ion Torrent, could analyze a person’s genome for $1,000. He’s now supplying CMU researchers with vast amounts of genome data from patients at Yale’s and Baylor University’s medical centers. The researchers hope to tap the power of computers to discover patterns and the molecular changes underlying complex diseases such as diabetes.
A recent New York Times article touched on the difficulty of bringing genome sequencing into routine clinical practice: “That long-awaited threshold ($1,000) excludes the cost of making sense of that data, which is becoming a bigger part of the total cost…” The article quoted Mark Gerstein, a Yale University professor of biomedical informatics, who said, “The real cost in the sequencing is more than just running the sequencing machine. And now that is becoming more apparent.”
Eventually, the hope is that, with genetic information at their fingertips, doctors could advise patients on what diseases they are most at risk for, and maybe even fix the related defect before the disease process starts. And for those already with a disease, doctors could employ new kinds of drugs that target genetic variations at the root of disease, and do so with fewer side effects and lower costs.
Some of this promise is already happening. The widely prescribed drug Coumadin (Warfarin) can be dosed more accurately by looking at a patient’s genotype to see how fast, or slowly, he or she will metabolize the drug and avoid side effects that put many Coumadin takers in the hospital. Last March, U.S. regulators approved Benlysta, the first new drug for lupus in more than 50 years. The developer, Human Genome Sciences, used genetic analysis to find proteins that are a cause of lupus. Last year also brought about a new FDA-approved drug for lung cancer, the latest in a number of drugs based on genomics already on the market for leukemia, breast, colon and other cancers.
The mapping of the human genome got much of the spotlight, but the mapping of other genomes, such as those of viruses, parasites and even cancer tumors, are critical too. “Where we are headed in the not-too-distant future is the sequencing of the entire DNA of an individual cancer,” said Dr. Nancy E. Davidson, director of Pitt’s Cancer Institute and UPMC Cancer Centers. With this information, she explained, doctors can understand the genetic changes in a tumor and employ targeted treatment. Right now, she added, it costs about $10,000 to sequence an entire tumor but that cost is likely to come down.
Pitt is at the forefront of this effort. For example, its head and neck cancer team, under the leadership of Dr. Jennifer R. Grandis and working with Harvard researchers, reported the DNA sequences for head and neck cancers last summer in the journal Science. Pitt also is providing some of its extensive repository of well-preserved cancer tissues to a federally funded project known as The Cancer Genome Atlas, which seeks to sequence many cancers. (Cleverly, its TCGA initials are the four letters that represent the four basic chemical units of DNA.)
“I don’t think all of this will change the face of cancer treatment immediately,” said Dr. Davidson, explaining that cancer is many very different diseases that are extremely complex. But it will perhaps lead to breakthroughs so that cancer is less likely to be fatal, but rather a manageable, chronic condition. That day couldn’t come soon enough for Davidson, whose 53-year-old, non-smoking sister died last summer, two weeks after being diagnosed with lung cancer.
When Dr. Naftali Kaminski decided 14 years ago to focus his genomic research on idiopathic pulmonary fibrosis (IPF), an older colleague told him he was wasting his time. There was nothing science could do for an irreversible disease that turned lungs into sacs of scar tissue, slowly suffocating its victims.
Today, several drug trials for IPF are being tested, thanks to genomic and molecular research. “I’m pretty optimistic that we’ll start having drugs that will have some effect in the next couple of years,” said Dr. Kaminski, director of UPMC’s Dorothy P. & Richard P. Simmons Center for Interstitial Lung Disease and the Lung, Blood and Vascular Center for Genomic Medicine. “We and others have found some novel mechanisms including molecules and noncoding RNAs [part of the genome] that seem promising as drug targets.”
Genomic research has given researchers the ability to tease out those patients who have a makeup that gives them a protective edge against IPF. This makeup includes genes as well as proteins in their bloodstream. Some of these patients live longer than eight years, while 50 percent of IPF patients die within three years of diagnosis. It may even turn out that some are able to rebuild lung tissue, something that so far has only been shown in mice. “Knowing who has this protective edge has very practical implications,” Dr. Kaminski said, “as the only effective therapy at this stage is lung transplant, which is not an ideal solution. So you could reserve transplant for those whose disease you know is going to progress quickly.”
Now, only IPF patients who are part of research studies are having their genetic and blood protein “biomarkers” identified, but Dr. Kaminski suspects that within a few years this will be a routine part of the workup for IPF patients. Also in a couple of years, he suspects laboratories will be able to offer a genetic test so family members of IPF patients would know whether they, too, have the genes associated with the disease. “Our success at the Simmons Center is a result of a unique collaborative environment. It is great when you can get clinicians, nurses, basic scientists and computational experts to work so tightly together as we do here. But it is even better when the patients become members of the team.”
Decades ago, the upper floors of Allegheny General Hospital’s North Side tower housed the operating theater. The state’s first heart valve replacement was performed there. Today, it is home to pioneering work of another kind, housing both the Center for Genomic Sciences and Gene Therapy Program.
Garth D. Ehrlich, Ph.D., oversees a team of researchers at the Center for Genomic Sciences. About five years ago, his center decided to focus on studying genomes of bacteria and viruses. Bacterial and viral infections kill more people than anything else. Infections, too, are what make chronic diseases, such as AIDS, chronic obstructive pulmonary disease, and even cystic fibrosis, deadly. A self-described gadget lover, Ehrlich makes sure his staff of 30 uses the cutting-edge tools to analyze genomes at lightning speed with as few errors as possible. He shows off the first-, second- and now third-generation of DNA sequencing computers. The latest SMRT (single molecule, real-time) technology, by Pacific Biosciences, can sequence an entire human genome in a few days.
“This technology enables the complete sequencing of a bacterial genome in about two to three hours at a cost of about $100,” said AGH spokesman Dan Laurent. It is only being used for research now, but “ultimately will usher in the era of personalized medicine” by making human genome sequencing a conventional diagnostic test.
Down the hall, Ehrlich shows off another gadget, the Ibis T5000. In six hours, this freezer-sized device can pinpoint the type of infection among thousands of strains of bacteria and fungi. Still only being used for research, it is showing very “promising results in clinical trials.”
A few floors away is the Gene Therapy Program, based on using normal genes to supplement or replace defective genes, or to bolster normal functions, such as immunity. “What if we can fix a disease before it starts? This is a big deal to me,” said program director Michael Passineau in a November lecture. “I’m a relatively early-career scientist. I’m going to watch this happen in my lifetime.” Passineau, however, also cited an example of why the field must proceed with caution. In 1999, an 18-year-old died four days after gene therapy for a liver condition. He suffered a massive immune response triggered by the virus that was used to transport a gene into his liver cells.
The case illustrates a major hurdle—evading the immune system. Until now, scientists have relied mostly on viruses to transport corrected copies of genes into a cell’s DNA. Viruses are adept at carrying a gene into a cell, but the immune system attacks them. Passineau may have found another way into cells. Using a once-obsolete ultrasound machine, he blasts gene drugs through cell membranes.
This work holds promise, however, only for certain parts of the body, such as salivary glands, which the immune system watches, but not as closely as some other parts of the body. “If you can’t carry the gene drug through a cell membrane, you can blow a hole in it. If we do this right, the hole seals up in a second or two and doesn’t seem to cause long-term damage to the cell.”
When it comes to tampering with genes and a person’s immune system, it’s important to take baby steps, Passineau says.
In 2009—just 10 years after the death of the 18-year-old—gene therapy was named runner-up breakthrough of the year in Science magazine. The article cited three promising clinical trials, including one at the University of Pennsylvania. As part of the trial, a boy with a genetic disease that causes blindness tried to navigate an obstacle course. In a video that Passineau shared during his recent genomics lecture, the boy clearly couldn’t see well enough to navigate the course. That same day, a surgeon injected a corrected copy of a gene into the boy’s eye. The boy tried again and easily succeeded.
“This is gene therapy. We have seen what looks like a miracle,” Passineau said, but quickly added: “Many of us in the field, myself included, believe this won’t last forever, maybe a year or two… But when you see something like this, anything seems possible. It’s important to remember, though, that clinical implementation is never as simple as we wish it was. We have many hurdles to overcome before we can call this disease, or others, ‘cured’ by gene therapy, but we will get there.”