Quantum Couple
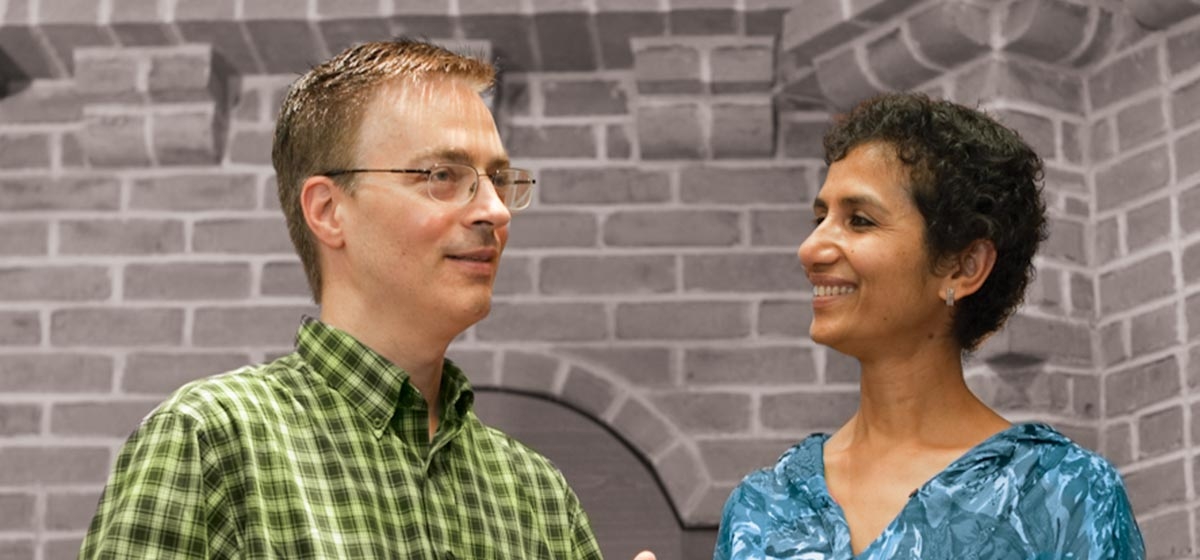
He grew up in Manhattan; she, in Patna, India. Both were taken with the way physics accounts for the world around them. After completing undergraduate studies at Harvard and the Indian Technology Institute, respectively, they met as first-year physics doctoral students at the University of California, Santa Barbara. Upon arrival she found herself the sole woman among 36 male classmates.
Searching for a female companion, she pored over the class roster. A first and last name ending in the letter Y caught her attention because in her native India, Y is a female suffix. She tracked down the classmate only to find that it was just another male colleague. But despite her initial disappointment, fortune smiled on her. The classmate with the Ys turned out to be Jeremy Levy, the man Chandralekha Singh would marry 18 months later.
Today they are professors of physics at the University of Pittsburgh and live with their teenage sons, Akesh and Ishan, in Schenley Farms, adjacent to Pitt. Jeremy explores the far reaches of quantum computing, one of the most challenging and elusive disciplines in all of academia. Chandralekha’s (chaun-dra-lay-kah) experiments take place in the college classroom where she devises innovative ways to teach burgeoning physicists how to think about the infinitesimally small and intractably weird world of quantum mechanics—the rules of how things smaller than atoms work.
A handsome couple to be sure, they can’t help but chuckle at nerdy physics jokes. Chandralekha tells one about Niels Bohr, one of the founding fathers of quantum mechanics, who is stopped for speeding by a highway patrolman. The trooper inquires, “Do you know how fast you were going?” Bohr replies, “No officer, but I do know exactly where I was.” The couple responded to my blank expression with kindly patience. The joke is funny to quantum physicists, they explained, because it puts the man who gave us the orbital model of the atom in the mundane position of trying to beat a speeding ticket by invoking one of quantum mechanics’ most sacred mysteries and fundamental truths: You can know either the speed or the position of a quantum particle, such as an electron, but not both at the same time.
In a similar vein, Jeremy related a story about teasing his 13-year-old son, Ishan, who has managed, over a period of months, to memorize the first 500 decimal places of pi. He told his son that because pi is an infinite number, he, Jeremy, could easily reel off 500 digits of pi by simply naming any random sequence of numbers, reasoning that any string of numbers must necessarily be found an infinite number of times in an infinite number such as pi. The only catch is he cannot say precisely where his sequence might appear.
“It’s a math joke,” Jeremy explained sheepishly, as I forced a smile to conceal my befuddlement. “It’s about infinity,” he added. “It’s a different sort of knowledge.”
Such different sorts of knowledge comprise the bulk of Jeremy and Chandralekha’s reality, which is laden with such notions as the well-established fact that observing a quantum particle changes it—not just optically or metaphorically, but physically; or that an electron can be in two places at one time—not very close by or in rapid succession—but in two completely different places at the very same instant; or that electrons can “tunnel” through solid objects; or that two unconnected subatomic particles can become entangled at long distances and communicate with each other.
Despite the impenetrably obscure nature of their chosen field of interest, Jeremy and Chandralekha each exhibit gifts for bringing the world of quantum physics within the grasp of mere earthlings. She demonstrates the effects of angular momentum with rotating bicycle wheels and spinning figure skaters. He uses a child’s Etch-A-Sketch as a model for his sketched oxide single electron transistors.
Jeremy’s innate appreciation of physics is evinced by his enthusiastic assertion that everything around us is based on quantum physics, from two-by-fours to cell phones. But beyond its ubiquity, quantum mechanics holds very serious consequences for computers and, therefore, all of us.
Although they are sure to differ from today’s computers in both the materials used to create and store data as well as in the processes used to operate on them, once quantum computers make the transition from laboratory bench to the real world, the most important difference will be speed. Today’s computers use the physical on/off states of microscopic switches—transistors—to process the most basic information possible: binary data bits (on/off, yes/no) by means of sets of logical arguments or algorithms executed on PCs, laptops and smart phones. In contrast to today’s computers, quantum computers are expected to use physically infinitesimal and computationally much more flexible bits of information called Q-bits, which will enable the compression, consolidation, multiplexing and acceleration of advanced computing operations. The result will be a computer with heretofore unimaginable speed.
Such an advance in processing speed is likely to have powerful consequences for Internet data security. Because the strength of Internet security is based upon the length of time it would take the most powerful computer to crack a given encryption code, a vastly faster computer could change the game forever. Experts estimate that today’s most secure encryption codes would take longer than the age of the universe to crack with today’s computers. Since the universe has been around for more than 13 billion years, we’re in good shape as of right now. However, a quantum-speed computer could change things quickly by cracking any imaginable encryption code in short order, including those employed for top secret government communications and—closer to home‚ your personal financial transactions. Clearly, whoever builds the first quantum computer will win the information technology sweepstakes.
In an effort to get there first, Levy (short e) is focusing his efforts on developing a transistor the size of a single electron. He begins with a layered pair of exotic materials that act as electrical conductors when they are four or more atomic layers thick, but serve as insulators at three or fewer. In a process that resembles a child’s Etch-A-Sketch, Levy “writes” and “erases” nanoscale circuits, roughly 15 atoms wide, by adding and removing electric charges on the top surface of this new double-layered material using the ultra-fine-tipped electronic probe of an atomic force microscope (AFM). By drawing a pair of intersecting circuits at right angles and zapping the intersection with the AFM tip, Levy creates a tiny, 1.5 nanometer insulated trap in the all-but-nonexistent space where the layers meet. Inside the trap, a group of negatively charged electrons become a synthetic atom known as a quantum dot. The remaining intersecting lines extending from the quantum dot become four leads which act as nanoscale circuits. With a subsequent, positive zap, single electrons can be coaxed into tunneling through the insulating walls of the trap and into the leads. Then with an oppositely charged zap, they tunnel back into the quantum dot again. Because these trapped electrons can control electric current flowing into the leads, much the way a conventional transistor controls current into and out of its circuits in today’s computers, the result is a transistor that is several thousand times smaller than today’s. Scaling things down even further, Levy is working on ways of using the direction of an electron’s spin (clockwise or counterclockwise) as a data bit—a Q-bit—the holy grail of quantum computing.
Although Jeremy and Chandralekha make understanding quanta look easy, for many students, the different sort of knowledge demanded by quantum topics stubbornly resists comprehension. In response, Chandralekha focuses her efforts on devising strategies to effectively teach students how to think about quantum mechanics, before actually teaching them the details of the subject. She begins with what students already know and builds on that. For instance, she gives students a real-life, long-term memory of the effect of angular momentum on spinning bodies as various as orbiting electrons and collapsing neutron stars by sitting students in a swivel stool and spinning them while they hold a dumbbell in each hand. As they pull the dumbbells into their bodies, they spin faster as the energy of motion moves nearer the center of their bodies, just like figure skaters, subatomic particles and celestial bodies. It’s a practical lesson students can apply to their theoretical studies, in addition to being one they will remember throughout their careers.
In 2010, Levy was awarded a $7.5 million grant from the United States Air Force to develop a quantum computer. Then in September 2011, Levy and Singh were awarded a $1.8 million grant from the National Science Foundation and the Nanoelectronics Research Initiative of the Semiconductor Research Corporation to bring a new kind of computer out of the lab and into the real world.
In addition to Levy’s innovation research, the grant includes funding for Singh’s educational research. In collaboration with colleagues, she is developing a new education program (“OnRamp”) aimed at lowering the steep and treacherous learning curve for early stage quantum physics researchers. At the summer 2012 meeting of the American Association of Physics Teachers, Singh was awarded the association’s Distinguished Service Citation for her pioneering research in the teaching and learning of quantum mechanics.
The couple’s work promises to lead to a new paradigm of computing. But unlike today’s computers, which are based on “0”s and “1”s, this one started with a couple of Ys.